Rift Valley fever: Condensed Version
Introduction and history
Rift Valley fever (RVF) is a peracute or acute disease that mainly affects domestic ruminants and humans caused by a mosquito-borne RNA phlebovirus. In domestic animals, the disease is most severe in sheep and goats, resulting in high mortality in new-born lambs and kids and high percentage of abortion, particularly in pregnant sheep and goats . The infection in humans is usually associated with mild to moderate febrile illness (i.e. fever, myalgia, arthralgia, lethargy), but can progress to severe sequelae including ocular lesions and loss of vision, encephalitis, a haemorrhagic disease with necrotic hepatitis and a high case fatality rate in a minority of patients. Humans usually become infected from contact with virus-contaminated tissues and body fluids from infected animals, but mosquito bites can also transmit the virus. Outbreaks of the disease tend to occur when particularly heavy rains favour the breeding of competent mosquito vectors.
Since original isolation in Kenya, RVF virus (RVFV) has been contained for decades within the African continent, but in the last 30 years emerged outside its historic boundaries including crossing significant geographic barriers such as the Sahara desert into Egypt in 1977, the Indian Ocean to Madagascar in 1991, and the Comoros Islands in 2007, and the Red Sea to the Arabian Peninsula in 2000/01.
The first time that RVF was recognized outside eastern and southern Africa was during 1977/78, when a major epidemic occurred along the Nile Delta and Valley in Egypt, causing an unprecedented number of human infections and deaths, as well as numerous deaths and abortions in sheep and cattle and some losses in goats, water buffalo (Bubalus bubalis) and camels. Estimates of the number of human cases range from 18 000 to more than 200 000 with at least 598 deaths occurring from encephalitis and/or haemorrhagic fever.
The first known excursion of RVFV beyond the continent of Africa occurred in Madagascar in 1979 when the virus was isolated from mosquitoes and a laboratory worker, but no naturally occurring livestock infections were recognized until 1990/91 and 2008/09 when widespread disease in livestock and humans was reported.
For the first time RVF was reported in livestock and humans in the Islands of Comoros from 2008/11 and Mayotte in 2008 and 2018/19.
Both legal and illegal trade of live livestock including camels from Sudan, Mauritania and other countries in that region may contribute to the spread of RVFV to North African countries. It raised concerns for further spread of RVFV to closest Asian (particularly Saud Arabia) and other parts of the world.
Since the virus can be transmitted by a wide range of mosquitoes, and livestock circulate sufficiently high levels of virus to infect mosquitoes, many parts of the world would probably be receptive to the virus. A recent (2020) European Food Safety Agency (EFSA) Opinion publication stated that the risk of introduction of RVFV into the EU Member States through the movement of infected animals is very low, because of strict EU policies for the introduction of live animals from non-EU countries. Similarly, the risk of RVFV entry into the EU through infected vectors was considered very low or low, ,but the probability of the establishment of RVFV transmission, once introduced, was considered to vary from moderate to very high.
Aetiology
Taxonomy and molecular biology
Rift Valley fever virus is a member of Phlebovirus genus, family Phenuiviridae of the order Bunyavirales. The viral particles are spherical, 80 to 120 nm in diameter, and consists of an envelope and a ribonucleocapsid (RNP). It has a three-segmented, single-stranded, negative-sense RNA genome of about 12 kb comprised of large (L, 6.4kb), medium (M, 3.8kb) and small (S, 1.6kb) segments.. The M segment encodes four proteins in a single open reading frame: the precursor to the structural glycoproteins Gn and Gc and two non-structural proteins designated NSm1 and NSm2.
Functions and role of RVFV-encoded proteins
The glycoproteins mediate cell receptor recognition, virus entry by binding to specific cell surface receptors(s) and virions escape from infected cells. Both Gn and Gc likely contribute to virion assembly and interact with N protein. These glycoproteins induce the production of neutralizing antibodies, which play an important role in protection of animals against reinfection with the virus.
The N protein seems not be involved directly in pathogenesis, but it is essential for virion capsid formation, interacts with glycoproteins, and is involved in transcription and replication. The N protein is the most abundant protein in phlebovirus-infected cells and is strongly immunogenic, but it does not elicit neutralizing antibodies. However, immunization with recombinant N protein induces a partial immune protection in animals.
The S-segment encoded NSs protein is the main virulence factor. Removal of the NSs gene results in attenuation of RVFV, which has been demonstrated in naturally attenuated and in recombinant viruses. Since the NSs protein is the major virulence factor by antagonizing IFN responses, the direct attenuation of NSs function potentially limits virus replication due to a lack of inhibition of the primary host immune response.
The NSs and NSm genes were shown to play a role in RVFV replication in mosquito vectors. RVFV lacking NSs and NSm failed to infect Aedes aegypti, and in Culex quinquefasciatus infection rates were lower than for wild-type virus. The double deleted viruses might represent an ideal safe vaccine due to their inability to efficiently infect and be transmitted by mosquitoes.
The evolutionary history, dispersal, and genomics of RVFV are discussed below (see Epidemiology).
Epidemiology
Vector ecology and virus transmission
Multiple risk factors influence the extent (both in time and space) of re-emergence and expansion of RVF outbreaks in endemic regions and their emergence in previously RVF-free areas. These include, environmental conditions, susceptibility and immune status of host animals (herd immunity), vegetation density, climate change, trade of live livestock, animal movement and distribution of vectors . Current data suggest that more than 50 mosquito species, many of which with global distribution, can potentially act as vectors of RVFV.
The outbreaks of RVF that occurred in North and West Africa (e.g. Egypt, Mauritania and Senegal) in recent years differed in many respects from the pattern of disease which occurred in sub-Saharan Africa: in the former, outbreaks occurred independently of rainfall in arid countries, apparently in association with vectors that breed in large rivers and dams. It is possible that a proliferation in the construction of farm and river dams (e.g. along the Nile and Senegal rivers) facilitated the spread of RVFV. In contrast to the outbreaks of RVF in the drier parts of North Africa, epidemics in eastern, central and southern Africa have usually been associated with above average rainfall at irregular intervals of 5 to 15 years or longer. It is clear that the ecology of RVF is a prime example of how climate change and human-induced landscape alterations can impact the emergence and re-emergence of arbovirus diseases such as RVF.
The flooding of dambos (also refer to as broad vleis) or pans in sub-Saharan Africa and the humid weather conditions prevailing in epidemics favour the breeding not only of the floodwater aedine mosquitoes but also of non-aedine mosquitoes that serve as epidemic vectors, as well as other biting insects that are potential mechanical transmitters of RVFV. Infected livestock circulate high levels of virus and mechanical transmission of infection by mosquitoes, midges, phlebotomids, stomoxids, simulids and other biting flies is thought to play a significant role in epidemics.
Infection rates in vector populations may be quite low even during epidemics, usually below 0,1 per cent, but enormous numbers of aedines emerge from flooded dambos or pans and vertebrates are subjected to high mosquito biting frequency. Infected livestock and wild herbivores serve as a source of virus for mosquitoes and once infection is amplified in them, secondary or epidemic vectors such as Culex spp. and anopheline mosquitoes and other biting arthropods may become involved in transmission.
Different species of the Aedes and Culex genera are the main biological vectors of RVFV.
In contrast to aedine mosquitoes, Culex spp. deposit their egg rafts on the water surface, where, depending on the temperature, they hatch within two or three days.. Culex theileri is widespread, occurring throughout the east and southern African region and the Mediterranean region, extending into the Northern Oriental region.It is moderately ornithophilic, but also feeds on a wide range of other hosts such as goats, cattle, sheep and humans and serves as an arboviral bridge vector.
Although contagion has been demonstrated on occasion under artificial conditions, non-vectorial transmission is not considered to be important in livestock, as opposed to humans.
A high degree of herd immunity develops in locations where infection is most intense, as judged by high morbidity, mortality and antibody rates, and it can be surmised that this must be one of the factors that contributes to the abatement of epidemics. Nevertheless, the large numbers of animals that were on occasion vaccinated during outbreaks of RVF must ultimately have contributed significantly to the abatement of epidemics.
Apart from aedine and culicine mosquito vectors in southern eastern and central Africa Ae. caspius, Cx. pipiens, Cx antennatus and Cx. perexiguus have been reported as the main potential vectors during RVF outbreaks in Egypt while Ae. vexans and Aedes ochraceus serve as maintenance vectors and Ae. dalzieli and Cx poicilipes as possible secondary vectors in West Africa and Saudi Arabia. Culex poicilipes and Cx. tritaeniorhynchus, were also important vectors of RVFV in the Saudi Arabia outbreaks.
According to EFSA Opinion and other publications. the following potentially competent RVFV vectors are present in EU countries: Ae. vexans, Ochlerotatus caspius, Ochlerotatus detritus, Cx. pipiens, Cx. theileri, Cx. perexiguus, Cx. antennatus, Cx. ritaeniorhynchus, and Ae. albopictus. Of these Ochlerotatus caspius and Cx. pipiens are considered to be the most competent vectors. Culex pipiens (the main vector during the RVF outbreaks in Egypt in 1977/78) and Ae. albopictus are widespread in European countries) and competent to transmit RVFV. Few vector competence studies have been done on European mosquito species.
Virus maintenance in the inter-epidemic period
While the ecological drivers of most RVF outbreaks are relatively well understood, the central enigma that remains in the epidemiology of the disease concerns the fate of the virus during the inter-epidemic periods (IEP).
Surveys and laboratory studies have failed to prove that the virus is maintained in transmission cycles in birds, monkeys, baboons or other wild vertebrates, although it was felt at that time that wild ruminants could play a role similar to their domestic counterparts in areas where they predominate.
Although RVFV was isolated from unfed male and female Ae. mcintoshi mosquitoes (reported as Ae. lineatopennis) hatched in dambos on a ranch in Kenya during IEPs in 1982 and 1984, the role of vertical transmission in Aedes spp. in the maintenance of RVFV in the IEP has been poorly studied. Recently vertical transmission of RVFV in colonized Cx. tarsalis mosquitoes has been reported in the laboratory.Specifically, it is not known if this transmission mechanism alone is sufficient to maintain RVFV endemicity, or if a continuous low-level virus amplification in domestic animals and wildlife is required to maintain the virus in the environment during IEPs. Numerous serological studies showed that there is continuous cryptic low-level virus transmission of RVFV during IEPs ,without noticeable outbreaks of disease or clinical cases, particularly in less susceptible domestic livestock such as cattle, goats, camels as well as wildlife in different African countries (e.g., South Africa, Mozambique, Sudan, Kenya, ) and would seem to be important in the maintenance of RVFV. Rift Valley fever virus transmission during IEPs has also been reported in humans in Tanzania, Kenya, Gabon and Botswana.
Rift Valley fever virus activity during the IEP in wildlife, certain domestic livestock and humans highlights the importance of a continuous cryptic endemic transmission cycle and virus evolution in suitable epidemiological/ecological settings. Clinical signs in these susceptible species are often mild or subclinical and are either underreported or misdiagnosed.
The susceptibility of different livestock species and African wildlife species are discussed below (see Clinical signs).
Evolutionary history, dispersal, and genomics of RVFV
The evolutionary history of RVFV has been influenced by dramatic changes in the environment and land use throughout Africa in the past 150 years. Over this time span multiple levels of virus evolution have occurred, ranging from the macroscopic geographic translocations of virus genotypes across Africa to the molecular point-mutations and genome segment reassortment events. Genomic reassortment or homologous recombination are both potent mechanisms to generate genetic diversity and can eventually drive the emergence of novel RVFV variants. ittle evidence of homologous recombination among RVFV has been reported.
As a consequence of the expanding genome sequence database, the number of identified lineages of RVFV has increased from three in early analysis to 15 lineages (designated A-O) in the recent publication by Grobbelaar et al. Lineage A contains isolates from North Africa, lineages B-M and O contain isolates from east, central and southern Africa, and lineage N groups isolates from West Africa.
RVFV displays low genetic diversity. Irrespective of the genome segment analysed, the genetic diversity is approximately 4 per cent at the nucleotide and 1 per cent at the protein coding levels. Nevertheless, the minor nucleic acid and deduced amino acid differences that have been reported could account for differences in pathogenicity.
While no distinctive mutually exclusive correlation of virus genotype, virulence in livestock and humans, or geographic location can be observed with RVFV, virus isolates from one area tend to cluster together within each lineage, but virus genetic variants with distant origins are found within different lineages, providing evidence of widespread dispersal of RVFV throughout Africa. The magnitude of this long-distance and repeated translocation can be found by the monophyletic linkage of isolates from regions as distant as Egypt, Madagascar, and Zimbabwe or Kenya, Mauritania, Burkina Faso, Zimbabwe, and South Africa. The strong phylogenetic linkage (Figure 1) of virus strains from distant geographic locations suggests that the movement of infected livestock and the dispersal of mosquitoes may account for the spread of RVFV throughout continental Africa, Madagascar, and the Arabian Peninsula. On the other hand, could it be that these virus strains have been present in these distant geographic locations and only now detected with the availability of improved molecular techniques?
Although transportation on some routes could take a long time in relation to the course of the infection, RVFV antigen can persist particularly in the spleen, for up to 21 days after infection. Humans slaughtering or handling the tissues of such animals could have become infected and possibly served as the amplifying hosts for the infection of mosquitoes, since the putative vectors in Egypt include Ae. caspius, Cx. pipiens, Cx. attenuatus and Cx. perexiguus, which are known to feed on livestock and humans.
Likewise, the results of phylogenetic studies demonstrated that the RVFV detected on the Arabian Peninsula in 2000 was closely related to the virus that had caused the 1997/98 epidemic in East Africa. As stated above it is likely that infected animals were imported during the large 1997/98 epidemic in East Africa, and that infection smouldered until heavy rains precipitated the epidemic in 2000. Travelling time for animals exported from infected areas can be as little as 48 hours, well within the usual incubation period of the disease.
Results from phylogenetic studies in Kenya indicate ongoing RVFV activity and evolution during the inter-epidemic period and highlight the importance of a cryptic endemic transmission cycle that allows for the establishment of RVFV endemicity and to precipitate explosive outbreaks.
Host susceptibility
The susceptibility of livestock, wildlife and humans is discussed below (see Clinical signs)
Pathogenesis
Given the role of NSs as a major virulence factor for RVFV, variability within its amino acid sequences could possibly account for the phenotypic differences between different virus strains.
The pathogenesis of RVF encompasses the spread of virus from the initial site of replication to critical organs such as spleen, liver and brain, which are either damaged by the lytic effects of the virus or immunopathological mechanisms, or else there is recovery mediated by non-specific and specific host responses. By analogy with the course of events believed to follow natural infection with other arthropod- borne viruses, it can be assumed that there may be an initial transient viraemia that of too low an intensity to be detectable or, more likely, that virus is conveyed from the inoculation site by lymphatic drainage to regional lymph nodes where there is replication and spill-over of virus into the circulation to produce primary viraemia. This in term leads to systemic infection with intense viraemia that results from release of virus following replication in target organs.
In in vitro cultures, RVFV replicates in cells derived from virtually all tissues except primary macrophages and lymphoblastoid cell lines, yet in intact animals macrophages are infected. There is also selectivity for certain other tissues, as indicated by the demonstration of viral antigen in rats by immunofluorescence in littoral macrophages of lymph nodes, most areas of the spleen except T-dependent periarteriolar sheaths, foci of adrenocortical cells, virtually all cells of the liver, most renal glomeruli and some tubules, and scattered small vessel walls, as well as in necrotic foci in the brains of individuals with the encephalitic form of the disease. Immunohistochemistry staining of tissues of naturally infected adult sheep with RVFV showed positive antigen staining in a variety of cells including hepatocytes, adrenocortical epithelial cells, renal tubular epithelial cells, macrophages, neutrophils, epidermal keratinocytes, microvascular endothelial cells and vascular smooth muscle. The results of titration of infectivity in organ homogenates indicate that liver and spleen are the major sites of virus replication.
Rift Valley fever virus, which attaches to receptors on susceptible cells, is internalized by endocytosis and replication occurs in the cytoplasm. The non-structural NSs protein synthesized during replication enters the cell nucleus and forms the filamentous intranuclear inclusions seen histologically in RVF-infected tissues.
Viraemia may become demonstrable in lambs less than one week old within 16 hours of peripheral infection with small doses of RVFV, and persists for the duration of the illness, which may terminate fatally within 36 to 42 hours. In older sheep, goats and cattle viraemia becomes demonstrable one to two days after infection and persists for up to seven days, usually being most intense on the second to fifth days after infection. Maximum titres of viraemia recorded were 1010.1 MIPLD50/ml (mouse intraperitoneal 50 per cent dose /ml) in lambs, 107.6 in sheep, 107.5 in calves, 108.2 in kids, and 105.6 in goats, although individual animals may fail to develop demonstrable viraemia. Virus has been shown to persist in visceral organs of sheep, particularly spleen, for up to 21 days after infection. Viraemic titres of up to 108.6 MICLD50/ml have been recorded in human patients.
The peracute hepatic disease seen in mice given large doses of virus most closely corresponds with that which occurs in other extremely susceptible hosts such as new-born lambs and kids. Other age groups and species of farm animals and humans commonly experience benign infection, but as with rhesus monkeys, a variable proportion of adult sheep, cattle and goats and a small proportion of humans develop the fulminant hepatic form of the disease. In these hosts, however, hepatic necrosis may be a less dominant lesion than in mice, with evidence of lymphoid necrosis, vasculitis, nephrosis and haemorrhagic manifestations being relatively more prominent. Factors contributing to fatal outcome in the hepatic form of the disease include anaemia, shock and hepatorenal failure, with the kidney lesions possibly being as important as shock in producing anuria.
Lesions in target organs such as the liver in the acute disease are produced by the direct lytic effect of the virus on infected cells. As the primary lesions enlarge, numerous degenerated and necrotic hepatocytes and acidophilic bodies appear throughout the parenchyma. Ultimately there is massive necrotic hepatitis in which the residual primary foci can still be recognized as dense aggregates of cellular debris infiltrated by leukocytes.
The haemostatic derangement is most severe in the fatal hepatic syndrome, which manifests as a viral haemorrhagic fever with bleeding tendency and evidence of disseminated intravascular coagulopathy. Viraemia is intense and prolonged in individuals that develop the haemorrhagic syndrome, indicating that there is impaired clearance of viraemia and extensive dissemination of virus with attendant widespread tissue damage, and it is postulated that the critical lesions in the development of the haemorrhagic state are vasculitis and hepatic necrosis. Destruction of the antithrombotic properties of endothelial cells is thought to trigger intravascular coagulation, and the widespread necrosis of hepatocytes and other affected cells to result in the release of procoagulants into the circulation. Vasculitis and haemostatic failure result in purpura and widespread haemorrhages.
Ocular lesions may occur as a complication to RVF infection in humans, occasionally appearing at the time of the acute febrile illness but usually up to four weeks later. Reports of long term sequelae including permanent vision deficits and even blindness have increased the need for follow-up investigation of this potential outcome among human patients. The essential lesion appears to be focal retinal ischaemia, generally in the macular or paramacular area, associated with thrombotic occlusion of arterioles and capillaries, and is characterized by retinal oedema and loss of transparency caused by dense white exudate and haemorrhages. Sometimes there is severe haemorrhage and detachment of the retina.
Encephalitis occurs from one to four weeks after the acute febrile illness in a small proportion of human RVF patients. It is suggested that there is an immunopathological basis to the encephalitic syndrome in addition to the direct cytopathic effects of the virus.
Abortion is the typical, but not universal, outcome to infection of pregnant sheep, cattle and goats but it has also been reported in other animal species including camels and African and Asian buffalo. Abortions which occur soon after infection may be related to the febrile illness of the dam since there may be no evidence of foetal infection, but in most instances abortion follows foetal death with lesions in the foetus resembling those in new-born lambs, including extensive hepatic necrosis. However, the brain is also infected and virus or antigen can usually be recovered from foetal viscera and brain. Virus can be isolated from the placenta and viral antigen was demonstrated in sheep in epithelial (syncytial) cells of the maternal villi and foetal trophoblasts. Widespread necrosis of these cells may cause abortion. Septic metritis can occur as a sequel to retention of the placenta, which commonly follows abortion. In humans, an attempt to relate the occurrence of abortion to evidence of RVF infection in Egypt produced inconclusive results, but recently human miscarriage and vertical transmission events reported RVFV as the causative agent. (see Clinical signs).
Another potential foetal adverse event associated with RVFV has been demonstrated among foetuses of ewes inoculated with the live-attenuated Smithburn vaccine strain at 42 to 74 days of gestation may develop various brain and other anomalies such as porencephaly, hydranencephaly and micrencephaly as well as arthrogryposis, which in some cases is associated with hydrops amnii, prolonged gestation and dystocia. This vaccine strain was prepared by serial passage intracerbrally and peripherally in rodents and likely has retained high-level neurotropism in foetuses and should be used with caution in pregnant animals.
Clinical signs
Despite large amounts of data regarding individual species susceptibility, it should be noted that the pattern of RVF can differ across individual herds and flocks, between separate epidemics, and during the course of a single epidemic. For instance, disease may predominate in either sheep or cattle at a particular location or stage of an epidemic, and outbreaks, which occur after long intervals or in new locations, may be characterized initially by abortions and disease of adults, and at a later stage of the outbreak, by disease of neonatal or immature animals. It is understandable that abortion and disease of adult animals should be the major manifestations when an epidemic occurs in an immunologically susceptible herd or flock at a critical stage of the breeding cycle, or that disease of neonatal animals predominates after lambing or calving has taken place. Immune ewes confer colostral immunity on their lambs that is protective for up to five months.
Signs of the disease in domestic ruminants tend to be nonspecific, rendering it difficult to recognize individual cases of RVF. During epidemics, however, the simultaneous occurrence of numerous cases of abortion and disease in ruminants, together with disease of humans, tends to be characteristic of RVF. Factors that determine the morbidity and mortality associated with outbreaks of RVF include the virulence of the strain of virus and the susceptibility of the vertebrates involved.
Although new-born lambs are extremely susceptible and can be infected lethally with as little as 0,1 MIPLD50 of RVFV, it is frequently difficult to produce disease in non-pregnant sheep and cattle with high doses of ostensibly virulent virus.
Sheep and goats
In new-born lambs and goat kids the incubation period may be as short as 12 hours but is usually 24 to 36 hours. Onset of the disease is marked by the development of fever which may exceed 41 °C and which is often biphasic with a remission of 12 to 18 hours following the initial rise in rectal temperature. The fever subsides sharply a few hours prior to death. Affected animals are listless, disinclined to move or feed, and evidence of abdominal pain can be elicited. Respiration is rapid and often abdominal in terminal illness. The course of the disease is usually peracute and lambs rarely survive longer than 24 to 36 hours after the onset of the first signs of illness; many are simply found dead. In animals less than a week-old mortality is 90 per cent or greater.
Lambs and kids older than two weeks and mature sheep and goats are significantly less susceptible than new-born lambs to RVFV and may develop inapparent, acute or peracute disease. In the peracute disease animals die suddenly without exhibiting noteworthy signs of illness. Under field conditions most animals develop the acute disease. Following an incubation period of 24 to 72 hours there is fever of up to 42 °C that lasts for 24 to 96 hours, anorexia, weakness, listlessness and an increased respiratory rate. Some animals may regurgitate ingesta and develop melaena or foetid diarrhoea and blood-tinged, mucopurulent nasal discharge. Occasionally, animals may be icteric.
Reports on the progression of natural disease in goats are inconsistent. Goats are considered to be more resistant to the disease.
Pregnant animals may abort at any stage of gestation as a result of the febrile reaction and/or placental necrosis. Aborted foetuses are usually autolysed. Although there is no evidence that fertility is impaired after abortion, this is possible in instances where retained placenta and purulent metritis occur as complications to abortion. Mortality and abortion rates vary between and within epidemics, but in southern Africa mortality rates varying from 5 to 30 per cent and abortion rates of 40 to 100 per cent were estimated for sheep. In contrast, it was estimated that up to 60 per cent of sheep died and 80 to 100 per cent of ewes aborted on some farms during the Egyptian epidemic of 1977/78. Goats were said to be more resistant to the disease in Egypt, whereas mortality in goats was reported to be 50 per cent in Sudan in 1973 and the abortion rate was estimated to be nearly 100 per cent in Mauritania in 1987.
A range of anomalies of the central nervous system including porencephaly, hydranencephaly and micrencephaly, as well as arthrogryposis, and other defects in foetuses associated with hydrops amnii, prolonged gestation and dystocia may occur if ewes are inoculated with live Smithburn strain vaccine between about five and ten weeks of gestation. If ewes are inoculated before this stage there may be unnoticed early loss of the conceptus, while inoculation at a later stage may result in abortion, stillbirth or birth of immune or viraemic progeny. Teratology following such vaccination has been recorded in the progeny of up to 15 per cent of pregnant ewes in flocks, but on average it appears to affect less than 2 per cent of ewes and abortion probably occurs in less than 10 per cent of pregnant ewes.
Cattle
The disease in calves resembles that in lambs and sheep, with occurrence of fever, inappetence, weakness and bloody or foetid diarrhoea, but a higher proportion of calves may develop icterus. In fatal disease, death generally occurs two to eight days after infection. Estimates of mortality during epidemics range from less than 10 per cent for all ages of cattle to 20 per cent for calves, but a mortality rate of 70 per cent was reported in experimentally infected one-week-old calves.
Infection is frequently inapparent in adult cattle, but some animals develop acute disease characterized by a high fever of 24 to 96 hours’ duration, anorexia, staring coat, lachrymation, salivation, nasal discharge, dysgalactia and bloody or foetid diarrhoea. The death rate in cattle does not generally appear to exceed 10 per cent. As in sheep and goats, cows may abort at any stage of gestation, the aborted foetus usually being moderately autolysed.
Other domestic and wildlife animal species
Pigs, horses and donkeys
Data suggests that adult and young piglets are resistant to experimental infection but develop transient viraemia if a high dose of virus is administered. However, more recently antibody positive pigs were reported among animals sampled at abattoirs in Egypt in 2009.
Horses develop only low-grade viraemia following experimental infection.
Camels and camelids
Antibodies were detected in old-world camels (Camelus dromedarius) in a part of Kenya where abortions occurred during a RVF epidemic in 1962, and again during a survey following the 1978/79 epidemic. The full potential impact of RVFV on camelids became apparent when large scale die-offs and abortions in dromedary camels occurred in 2009/10 in Mauritania.
Although infection in camels is generally subclinical in mature animals, pregnant camels may abort at any stage of pregnancy and neonatal deaths have been reported in Egypt and Sudan.
Antibodies to RVFV in camels have been detected in many African countries: seroprevalence rates varied from 3 to 57 per cent.
Water or Asian buffalo
High seroprevalence of anti-RVFV antibodies, abortion rate of 12 per cent and mortality rate of 7 per cent were found in domesticated Asian water buffalo (Bubalus bubalis) during the 1977/78 epidemic in Egypt.
Wildlife species
Antibodies against RVFV have been detected in many African wildlife species including topi (Damaliscus korrigum), red fronted gazelle (Eudorcas rufifrons), dama gazelle (Nanger dama), scimitar-horned oryx (Oryx dammah), common reedbuck (Redunca redunca), African buffalo (Syncerus caffer), Dorcas gazelle (Gazella dorcas), Thomson’s gazelle (Gazella thomsonii), gerenuk (Litocranius walleri), lesser kudu (Tragelaphus strepsiceros), impala (Aepyceros melampus) sable antelope (Hippotragus niger), waterbuck (Kobus ellipsiprymnus), warthog (Phacochoerus aethiopicus), African lion (Panthera leo), giraffe (Giraffa camelopardalis), Burchell’s zebra (Equus burchellii), black rhinoceros (Diceros bicornis), springbok, (Antidorcas marsupialis), wildebeest (Connochaetes taurinus), African elephant (Loxodonta africana), kongoni (Alcelaphus buselaphus), bats (Micropteropus pusillus, Hipposideros caffer, Hipposideros abae), and waterbuck (Kobus ellipsiprymnus).
Abortion as a result of RVF was reported in the late 1990s in African buffalo that were kept in a boma at Skukuza in the KNP.
To date, few experimental infections of European wildlife have been reported. North American white-tailed deer (Odocoileus virginianus) were recently found to be highly susceptible to lethal disease characterized by severe clinical illness and high-titre viraemias.
Humans
Humans become infected from contact with infected tissues or from mosquito bites.The majority of RVF infections in humans are inapparent or a transient influenza- like illness. A minority of patients develop ocular lesions, encephalitis or severe hepatic disease with haemorrhagic manifestations.
After an incubation period of two to six days, the onset of the benign illness is usually very sudden and the disease is characterized by rigor, a fever that persists for several days and is often biphasic, headache, retro-orbital pain, photophobia, weakness, and muscle and joint pains. Sometimes there is nausea and vomiting, abdominal pain, vertigo, epistaxis and a petechial rash. Symptomatic improvement occurs in four to seven days in benign RVF and recovery is often complete in two weeks.
Hepatorenal failure, shock, and severe anaemia are the major factors associated with death.
Rare cases of miscarriage and stillbirth have been reported recently.
There are numerous reports of humans becoming infected while investigating the disease in the field or laboratory. Generally, persons who become affected are involved in the livestock industry, such as farmers who assist in dystocia of livestock, farm workers who salvage carcasses for human consumption, veterinarians and their assistants, and abattoir workers.
The putative vectors in the outbreaks in Egypt in 1977/78 included Ae. caspius, Cx. pipiens, Cx. attenuatus and Cx. perexiguus, which are known to feed on livestock and humanswhile the principal mosquito vectors of RVF in southern Africa tend to be zoophilic and sylvatic but it is thought that humans can become infected on occasion through transmission by Cx. theileri and Cx. zombaensis.
No outbreaks of the disease have been recognized in urban consumer populations and it is surmised that the fall in pH associated with the maturation of meat in abattoirs is deleterious to the virus.
Human infection presumably results from contact of virus with abraded skin, wounds or mucous membranes, but aerosol and intranasal infection have been demonstrated experimentally and circumstantial evidence suggests that aerosols have been involved in human infections in the laboratory, and in the field during epidemic. Low concentrations of virus have been found in milk and body fluids, such as saliva and nasal discharges of sheep and cattle.
Despite the intense viraemia that occurs in humans and the fact that virus has been isolated from throat washings, there are no records of person-to-person transmission of infection.
The ocular disease usually presents as a loss of acuity of central vision, sometimes with development of scotomas. The essential lesion appears to be focal retinal ischaemia, generally in the macular or paramacular area.
Probably less than 1 per cent of human patients develop the haemorrhagic and/or encephalitic forms of the disease. The haemorrhagic syndrome starts with sudden onset of influenza-like illness similar to the benign disease, but within two to four days there may be development of petechial rash, purpura, ecchymoses and extensive subcutaneous haemorrhages, bleeding from needle puncture sites, epistaxis, haematemesis, diarrhoea and melaena, sore and inflamed throat, gingival bleeding, epigastric pain, hepatomegaly or hepatosplenomegaly and tenderness of the right upper quadrant of the abdomen and deep jaundice.
Signs of encephalitis in humans may supervene during the acute illness, or up to four weeks later and include severe headache, vertigo, confusion, disorientation, amnesia, meningismus, hallucinations, hypersalivation, grinding of teeth, choreiform movements, convulsions, hemiparesis, lethargy, decerebrate posturing, locked-in syndrome, coma and death. A proportion of patients may recover completely, but others may be left with sequelae, such as hemiparesis.
Table 1.
Susceptibility of vertebrates to Rift Valley fever virus infection. (Information derived from sources cited in the text)
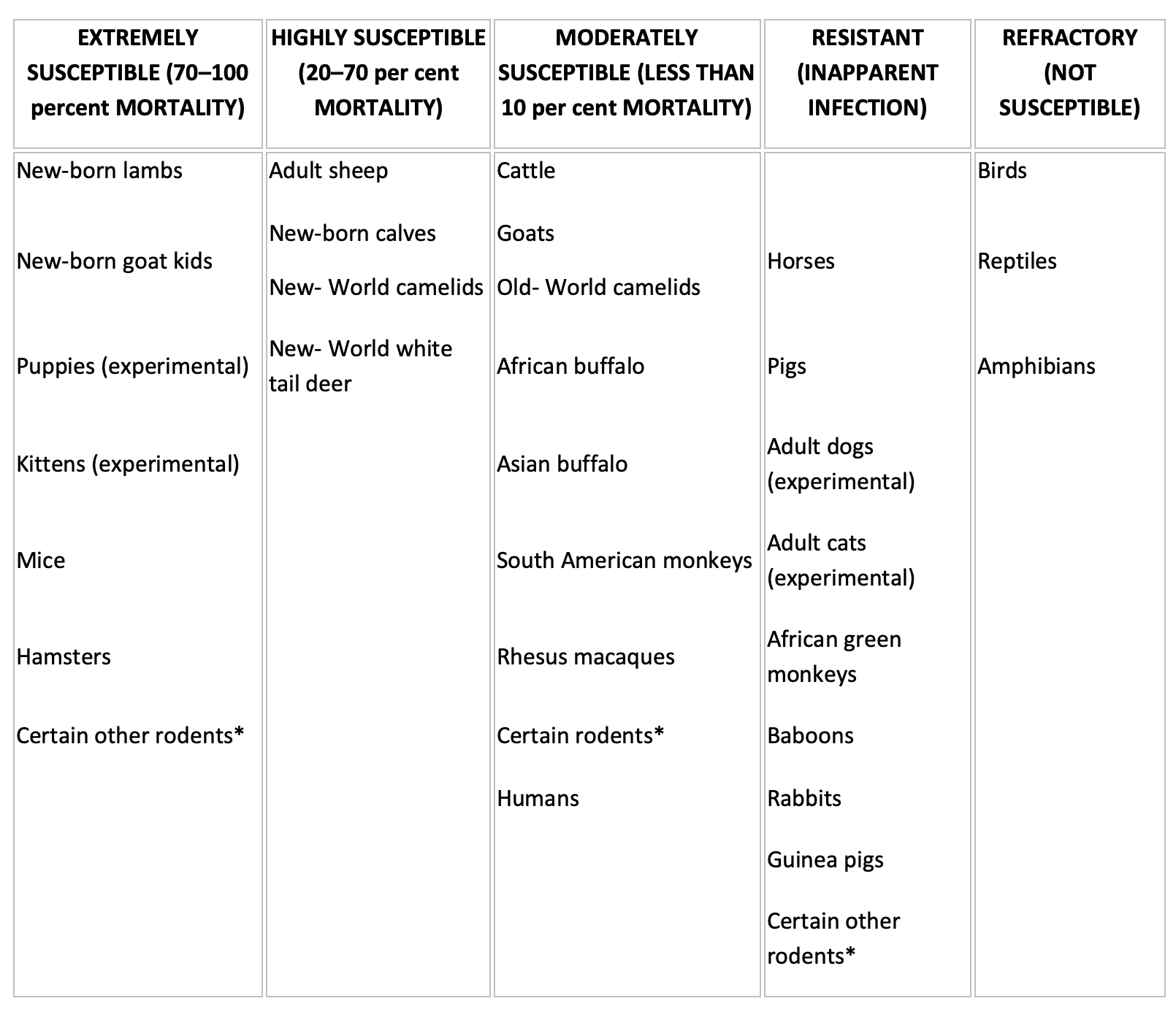
* See Pathogenesis
Pathology
The hepatic lesions of RVF are essentially similar in all domestic animals and humans, varying mainly with the age of the affected individual. The most severe lesions occur in aborted sheep foetuses and new-born lambs in which the liver is usually moderately to greatly enlarged, soft, friable and yellowish-brown to dark reddish-brown in colour with irregular congested patches and sometimes haemorrhages of varying size scattered throughout the parenchyma (Figures 2 and 3). Numerous greyish-white necrotic foci, 0,5 to 1,0mm in diameter, are invariably present in the parenchyma but may not be clearly discernible due to the discoloration of the organ. There may be oedema and haemorrhages in the wall of the gall bladder and hepatic lymph node. Icterus is evident in only about 10 per cent of affected new-born lambs because of the peracute course of the disease.
The hepatic lesions in adult sheep are generally not as severe or as widespread as in new-born lambs, but icterus may be more evident because of the less peracute nature of the disease. Pin-point reddish to greyish-white necrotic foci may be distributed throughout the parenchyma (Figure 4), and in a small proportion of sheep there are centrilobular haemorrhages and necrotic lesions which impart a mottled appearance (Figure 5) to the organ and render lobulation more distinct than normal.
Haemorrhages and oedema of the wall of the gall bladder are common, and the lumen may contain a blood coagulum or blood-tinged bile (Figure 6).
The livers of aborted cattle foetuses resemble those of lambs, while the livers of calves and adult cattle are swollen, friable, discoloured orange-brown, and sometimes show congested areas and haemorrhages in the parenchyma with accentuation of the lobulation. While only scattered, greyish-white foci of necrosis, 0,5 to 1,0 mm in size, are seen in the livers of adult cattle, numerous foci are usually discernible in calves.
The hepatic lesions in new-born lambs are almost invariably accompanied by numerous petechiae and ecchymoses in the mucosa of the abomasum, and its contents are dark chocolate-brown as a result of the presence of partially digested blood (Figures 7 and 8). The contents of the small intestine may be similar in appearance. Most mature sheep and cattle have haemorrhages and oedema in the abomasal folds (Figure 9), and sometimes copious amounts of free blood in the lumen of the intestines. In most animals the spleen is slightly to moderately enlarged, with haemorrhages in the capsule. Infarcts are occasionally evident on the edges of the spleen in adult sheep as dark blueish-red, circumscribed areas, 10 to 20mm in diameter.
Other changes include widespread subcutaneous, serosal (Figure 10) and visceral haemorrhages, mild to moderate effusion of fluid, often blood-tinged, into body cavities, congestion and oedema of the lungs, enlargement of the adrenals with small haemorrhages in the cortex, and nephrosis which may be particularly severe in mature sheep.
Hepatic necrosis is the most striking microscopic lesion of RVF in all domestic animals and humans. In aborted sheep foetuses and neonatal lambs, primary foci of necrosis comprising dense aggregates of cytoplasmic and nuclear debris, some fibrin and a few neutrophils and macrophages can be discerned against a background of parenchyma reduced by nuclear pyknosis, karyorrhexis and cytolysis to scattered fragments of cytoplasm and chromatin, with only narrow rims of degenerated hepatocytes remaining reasonably intact close to portal triads (Figures 11 and 12). Destruction of hepatocytes may be so marked that most of the normal architecture of the organ is lost, giving the liver almost the appearance of lung tissue (Figure 11). Intensely acidophilic cytoplasmic bodies which resemble the Councilman bodies of yellow fever are common, and rod-shaped or oval eosinophilic intranuclear inclusions (Figure 13) are found in about 50 per cent of affected livers. Mineralization of necrotic hepatocytes may be evident as small purplish-blue cytoplasmic granules (Figure14) in haematoxylin and eosin-stained sections in approximately 60 per cent of lambs, and bile casts are present in about 30 per cent of livers. Other noteworthy lesions in new-born lambs are pyknosis and karyorrhexis of lymphocytes in lymphoid tissues, cloudy swelling and hydropic degeneration of the epithelial cells of the convoluted tubules of the kidney and necrosis of some of the cellular elements in the glomeruli in ten per cent of lambs, and multifocal necrosis and haemorrhages in the adrenal cortex.
In older sheep, liver necrosis is not usually as diffuse as in neonates and tends to be multifocally distributed (Figure 15) throughout the parenchyma and icterus is more common than in lambs. The hepatocytic changes in these foci are similar to those described for primary foci of necrosis in lambs. However, occasionally centrilobular necrosis and haemorrhage are also evident. Severe nephrosis which occasionally occurs in mature sheep is characterized by marked tubular degeneration and necrosis, intracytoplasmic accumulation of proteinaceous droplets in tubular epithelial cells and presence of hyaline casts in tubular lumens, while haemorrhages, necrosis and deposition of fibrin and haemorrhage may be present in glomeruli (Figure 16). A few acute subcapsular infarcts are occasionally present at the edges of the spleen and the sinuses may contain accumulations of excess fibrin.
Primary foci of necrosis are particularly numerous and conspicuous in the livers of affected calves (Figure 17) and aborted cattle foetuses, and are sometimes accompanied by massive hepatic necrosis, presenting a pattern similar to that described for new-born lambs.
Marked centrilobular eosinophilic necrosis and haemorrhage, frequently extending to the middle of the lobule, occur in about 60 per cent of field cases of RVF. Massive necrosis involving almost all hepatocytes (Figure 18) occurs in approximately 30 per cent of livers of field cases, while focal necrosis occurs in the remainder. Other lesions in cattle are similar to those described for calves.
Varying degrees of lymphocyte necrosis have been described in the spleen and lymph nodes in sheep, cattle and humans.
Diagnosis
Suspicion of RVF should be aroused when heavy rains are followed by the occurrence of abortions in sheep, cattle and goats together with fatal disease, particularly in young and pregnant animals, which is marked by necrotic hepatitis and widespread haemorrhages. Frequently, there is also an influenza-like illness in farm workers and others with contact to infected animal tissues.
The usually regional nature across national borders of RVF outbreaks and involvement of multiple species requires an integrated One Health approach to outbreak investigation, diagnostics, and counter-measure responses. Due to the severe potential economic impacts of RVF outbreaks in animals, animal health professionals should familiarize themselves with the specific guidelines and requirements listed in the World Organization for Animal Health (WOAH) Manual for diagnostics and regulatory reporting requirements.
Field biosafety and biosecurity concerns for veterinarians and other health personnel
Rift Valley fever virus has proven on multiple occasions to be easily transmitted to people from infected animal tissues and body fluids in farm settings. If RVFV is suspected among livestock, standard biosafety and biosecurity protocols and procedures should be followed to avoid infection of animal health workers and other personnel involved in the collection of diagnostic specimens. At a minimum for on-site field investigations, gloves, eye/face protection, and dedicated field clothing and boots should be worn and thoroughly disinfected with appropriate disinfectants labelled for virucidal activity against lipid-enveloped viruses. Ideally, when handling suspected RVFV infected animal carcasses or materials, personal-protective equipment (PPE) and practices should be supplemented by disposable coveralls and respiratory protection such as properly fitted N95 respiratory masks. Health workers should consult their national agricultural and public health authorities for proper guidance related to PPE and safe sampling procedures relevant for local conditions and practices.
Work with live infectious virus, non-inactivated clinical specimen materials, and experimentally infected animals should be conducted in laboratories (i.e., BSL-3 or higher) with appropriate biosafety facilities and PPE practices.
Specimen collection
Specimens to be submitted for laboratory confirmation of the diagnosis include either EDTA, heparinized or clotted whole blood, plasma or serum of live affected animals or tissue samples, including liver, spleen, kidney, lymph nodes and heart blood of dead animals. Samples from aborted foetuses should include brain since this is usually less autolysed or putrified than viscera when obtainable. Placenta specimens, if available, can also be collected.
Specimens should be securely packaged and submitted at 4 °C to a suitable laboratory for isolation or detection of virus or demonstration of antibody. Where delay in getting specimens to the laboratory is unavoidable or the material has to be transported at ambient temperature, tissue samples can be preserved in glycerol-saline solution. Tissue specimens from the liver, spleen, lymph nodes and kidney should also be collected in 10 per cent buffered formalin for histopathological and immunohistochemical examination. In animals that survive the disease, paired serum samples, one taken during the acute illness and the other two to three weeks later, should be submitted for antibody tests.
Diagnostic assays
Molecular detection assays: The frontline diagnostic assays routinely employed in centralized diagnostic laboratories are nucleic-acid amplification tests such as real-time reverse transcription polymerase chain reaction (RT-PCR), quantitative real-time PCR, or whole genome sequencing. These detection assays require moderate laboratory sophistication and technology, that are routinely available in most regional diagnostic centres. Multiple broadly reactive assays have been described and are routinely used to rapidly and accurately identify RVFV genome in a wide variety of submitted specimens (e.g. blood, plasma, serum, homogenized tissues, and other fluids). Viral nucleic acid can readily be detected in serum and other tissues of infected humans and livestock, as well as mosquitoes, by RT-qPCR. and real-time reverse transcription-loop-mediated isothermal amplification assay.
Antigen detection assays: RVFV antigens can be detected by immunofluorescence and immunocapture ELISA assays. A safe sandwich ELISA (sAg-ELISA) has been developed for the detection of RVFV NP antigen in specimens inactivated at 56 °C for 1 h in the presence of 0.5% Tween-20 (v/v) before testing. The test can be used for the detection of RVFV NP antigen in human and animal sera, homogenates of liver and spleen tissues of domestic and wild ruminants, and mosquito homogenates. The lateral flow immunochromatographic test for the detection of RVFV NP in animal sera and fluids from aborted foetuses is a valuable diagnostic tool for onsite rapid detection of RVFV.
Virus isolation and culture: Long considered the gold-standard of virus diagnosis, this technique requires relatively high levels of biosafety and biosecurity and has become less favoured than molecular diagnostic assays. However, obtaining high quality virus isolates from field specimens is essential for further research work and development and should be considered where adequate laboratory facilities and trained staff are present. Rift Valley fever virus can be isolated readily in a variety of cell cultures including Vero, CER, BHK21, mosquito line cells, and primary calf, lamb and goat kidney or testis cells, or in suckling and weaned mice or hamsters inoculated intracerebrally or intraperitoneally. Cytopathic effect (CPE) may be evident one to five days after inoculation of cell cultures, but virus can be identified and the diagnosis accelerated by performing immunofluorescent (IF) tests on cultures at 24 hours or even earlier. It is always necessary to perform IF tests on inoculated mosquito cells since CPE is not readily apparent in them. More commonly, virus isolated in cell cultures is identified with reference antiserum by performing neutralization tests. Inoculation of suckling mice by the intracerebral route is used extensively for isolation of RVFV, and mice generally die two to five days after inoculation with field material.
The virus can be grown in and readily produces cytopathic effect and plaques in virtually all common continuous line and primary cell cultures, including Vero and BHK21 line cells, primary calf and lamb kidney or testis cells, the only major exceptions being primary macrophages and lymphoblastoid cell lines. The virus can be grown in embryonated chicken eggs and a variety of laboratory animals including suckling or weaned mice and hamsters inoculated by intracerebral or intraperitoneal routes. Lambs are extremely susceptible, but some strains of rat are resistant, as are rabbits, guinea pigs, horses, and chickens.
Infectivity of virus stocks is highly sensitive to temperature and storage conditions. Controlled studies of the Clone 13 vaccine strain revealed that virus particles retain infectivity in serum and can be recovered after prolonged (weeks to months) storage at 4 °C; however increased temperature rapidly degrades virus particles and infectivity is lost within hours at 56°C. The virus is very stable at temperatures lower than –60°C or after freeze drying. It is inactivated by lipid solvents, low concentrations of formalin, and is very sensitive to acidic conditions with infectivity rapidly lost below pH 6.8.
Microscopic pathology and immunohistochemistry: Histopathological lesions are very characteristic and, in particular, the liver lesions of new-born lambs and kids leave little room for doubt about the diagnosis of RVF. Virus antigen can be detected in tissues of infected animals by immunohistochemistry.
Antibody detection assays: Antibody to RVFV can be demonstrated by different assays including ELISAs, multiplex-bead based arrays, indirect immunofluorescence, and virus plaque reduction neutralization test (PRNT). All techniques except neutralization tests can be performed with inactivated antigens to enhance biosafety in the laboratory. Although regarded as a gold standard, virus neutralization assays are laborious, expensive, and require 5-7 days for completion. They can be performed only when a standardized stock of live virus and tissue cultures are available. Consequently, they are rarely used, and only in specialized laboratories equipped with high biocontainment facilities. Recently, a neutralization test based on an avirulent RVFV expressing an enhanced green fluorescent protein was developed and reported to be more sensitive than the classical neutralization test and safe to be used outside level 3 biocontainment facilities
Various ELISA formats have been developed and validated in recent years for specific detection of anti-RVFV antibodies in humans and animals, based on inactivated sucrose-acetone-extracted antigens derived from tissue culture or mouse brain. While they were shown to have high diagnostic specificity and diagnostic sensitivity compared to virus neutralization assays, the production of antigen for these assays also requires bio-containment facilities to limit the risk of exposure of laboratory personnel to infection. To address these problems, indirect ELISAs based on the recombinant NP and GP proteins have been developed for the detection of anti-RVFV antibodies in domestic ruminants, wildlife and humans. An ELISA platform based on recombinant NP and NSs proteins is able to distinguish infected from vaccinated animals. This diagnostic capacity is important for allowing moving of vaccinated animals, given the strict regulations for movement and export of animals.
Since a wide range of domestic and wild animal species are susceptible to RVFV, the development of inhibition and competitive ELISAs provide an additional advantage of allowing multi-species RVFV antibody detection using the same diagnostic procedure without requirements for species-specific conjugates.
Recent or current infection must be distinguished from pre-existing immunity and, conventionally, serological diagnosis of recent disease is confirmed by demonstrating seroconversion or a four-fold or greater rise in titre of antibody in paired serum samples. The introduction of the IgM-capture ELISA for detection of antibody to RVFV in humans and domestic ruminants, however, allows diagnosis of recent infection to be made on a single serum sample.
Results obtained in experiments with sheep indicate that neutralizing antibody may become demonstrable as early as three days following infection and by the fourth to sixth day antibody is detectable by ELISA. Antibody titres detected by ELISA are maximal from two weeks to approximately six months after infection. Thereafter ELISA titres decline over a period of several years, while neutralizing antibody tends to maintain a plateau level after an initial post-convalescent decline and probably remains demonstrable for the life of the individual. Kinetics of IgM and IgG responses were studied in sheep experimentally infected with wild-type RVFV and in sheep vaccinated with live-attenuated Smithburn strain of RVFV. IgM and IgG seroconversion was detectable 4-6 days post-infection with IgG antibody reaching the highest level on Day 21 and IgM antibody on Day 11 after infection. Compared to sheep infected with wild-type virus, the IgG and IgM responses in vaccinated animals were less rapid and antibody levels and especially those of IgM were lower. IgM antibody could be detected only in 12.5 per cent of infected sheep 72 days post-infection. The time dependent detectability of anti-RVFV antibody, and especially IgM, may be of significance for epidemic situations, where the disease stage may affect the outcome of an assay result and interpretation of the data. The only information available on the duration of the IgM antibody response following natural infection relates to 195 cattle monitored after the 1991 epidemic in Madagascar; less than 30 per cent had IgM antibody two months after infection was diagnosed, and all were negative by six months.
Establishing a definitive diagnosis in cases of teratology caused by the live modified RVF vaccine virus can be very difficult. Several viruses, other agents, and plant toxins can cause similar teratology. Foetuses may not be immunocompetent at the time that they undergo teratogenic infection and thus may not have virus or antibody at the time that the malformations become apparent, thus limiting diagnostic options for specific testing. Regarding serological diagnoses of new-born animals, those that are able to suckle acquire maternal IgG but not IgM antibody from milk ingestion. Compounding this, dams of affected foetuses will generally no longer have IgM antibody by the time that malformations become apparent. Identification of the teratogenic agent may need to rely heavily on risk-factor analysis and epidemiology to support a tentative aetiological diagnosis.
Differential diagnosis
Rift Valley fever and other arthropod- borne virus diseases tend to occur under the same climatic conditions.
Definitive diagnosis of RVF ultimately depends on virological and serological examination of appropriate specimens but the diseases can also be distinguished on the basis of the hepatic changes found in each.
Agents causing mortality associated with hepatic lesions, haemorrhages and/or icterus which may superficially resemble RVF in domestic ruminants include bacterial septicaemias such as pasteurellosis, salmonellosis (calf paratyphoid) and anthrax.
Sometimes abortion is the only sign of RVF in cattle and diseases, which must be eliminated by appropriate laboratory investigations, include brucellosis, leptospirosis, chlamydiosis, Q fever, and salmonellosis. In instances where teratology is involved in sheep, investigations should include Schmallenberg virus, bluetongue virus, Simbu-serogroup bunyaviruses, Palyam serogroup orbiviruses, Wesselsbron disease virus and other flaviviruses such as West Nile and Banzi.
Rift Valley fever belongs to a group of viral haemorrhagic fever(VHF) diseases with worldwide distribution, but their specific aetiological agent is usually restricted to a known endemic region depending on the presence of natural reservoirs or/and competent arthropod vectors. As for most VHFs, the non-specific clinical manifestations of RVF makes it difficult to diagnose clinically. Therefore, the differential diagnoses of RVF in humans include a broad spectrum of conditions especially when first cases are encountered during a yet unrecognized outbreak. These include malaria, rickettsial infections, Q fever, typhoid fever, dysentery, plaque, brucellosis, leptospirosis, meningitis, sepsis from bacterial infections, viral hepatitis, other VHFs such as Lassa fever, Crimean Congo haemorrhagic fever, Marburg disease, Ebola fever, and the haemorrhagic fever associated with the renal syndrome caused by hantavirus infections.. Rift Valley fever in humans should be suspected when there is a sudden outbreak of febrile illness with headache and myalgia in humans, in association with the occurrence of abortions in domestic ruminants and high mortality of young animals such as lambs and kids.
Control
Vector control
The large number of mosquito species that can transmit RVFV and the vast number of breeding sites, particularly after heavy rains, makes it difficult to control mosquitoes on a large scale. Mosquito control during outbreaks is in most instances thus not practical and is almost never applied. In the case of dairy farms, the milking sheds should be made mosquito proof, and perhaps Culicoides-proof, and monthly spraying of their interior walls should be carried out with a residual insecticide other than an organochlorine. Organophosphate insecticides, such as malathion, fenthion (Baytex), fenitrothion (Sumithion), or carbamates such as carbaryl (Sevin) and propoxur (Arprocarb), can be used.
One Health approaches, vaccination and therapeutics
The central role of particularly viraemic livestock as a risk factor for human infection highlights the need for comprehensive One Health control strategies. For the successful long-term control and management of RVF in endemic areas, a multifaceted approach is needed that can reduce the level of virus circulation and amplification among mosquito vectors, livestock, and ultimately humans. Targeting one of the key amplification components, namely livestock, of the RVFV epidemic cycle could eliminate some of the greatest risk factors for human infection while at the same time greatly improving the economic and nutritional well-being of livestock producers and animal product consumers. Critical for this control approach to be successful will be the ready availability of inexpensive and effective vaccines that can be used safely in livestock or humans regardless of pregnancy and age status.
Vaccines – historical and recent approaches
Vaccines have been an important component of routine management and emergency outbreak responses to RVF since the 1950s. Animal vaccination is the most effective control measure, preventing both human and livestock cases.
Wild-type RVFV, also referred to as hepato-, viscero-, or pantropic virus, has been attenuated to produce vaccine or candidate vaccine strains by serial passage in highly permissive systems such as suckling mice or cell cultures, by selection of small plaque variants and by chemical mutagenesis with 5-fluorouracil. The 5-fluorouracil mutagenized MP12 candidate vaccine virus has mutations in all three segments of the genome. The Smithburn vaccine strain was developed by serial intracerebral passage of a Ugandan wild-type virus in suckling mice until it lost its tropism for the liver and became neuroadapted, i.e. until it no longer killed mice following peripheral inoculation yet retained its intracerebral lethality and its immunogenicity. Clone 13, a naturally occurring and highly attenuated mutant obtained by plaque selection of clones from a human isolate of RVFV from the Central African Republic, proved to have a 549 nucleotide deletion comprising 69 per cent of the NSs protein gene in the S RNA segment of the genome. The thermostable RVFV Clone 13 vaccine (CL13T) has been developed via a selection of viable virus populations at 56⁰C from the culture supernatant of Vero cells infected with the original Clone 13 strain of the virus, and then lyophilized in the presence of a stabilizer.
Attenuated Smithburn strain and formalin- inactivated vaccines: The mouse neuroadapted Smithburn strain of RVFV is used at slightly different passage levels in laboratory host systems for production of livestock vaccines in South Africa and Kenya, while wild strains of RVFV are used for preparation of formalin-inactivated cell culture vaccines in South Africa and Egypt.
In contrast to the Smithburn vaccine, formalin-inactivated vaccines are safe for use even in pregnant animals, but they are expensive to produce and induce short-lived immunity, so that the administration of annual booster doses is necessary to ensure adequate protection.
The modified live Smithburn vaccine can readily be produced in large quantities, is inexpensive, and induces durable immunity in sheep six to seven days after a single inoculation, but in a proportion of pregnant animals it may cause abortions or teratology of the central nervous system of the foetus and hydrops amnii and prolonged gestation in the dam. It is advised that inactivated vaccines should be used in situations where it is considered necessary to immunize animals in countries where the presence of RVF has not been proven.
The Smithburn RVF vaccine induces a poor antibody response in cattle, and they should preferably be immunized with formalin-inactivated vaccine to ensure that cows are able to confer colostral immunity to their offspring. Cattle should receive a booster dose three to six months after initial vaccination, followed by annual boosters before the rains are due, since immunity only lasts about one year. Formalin-inactivated vaccine can also be used in pregnant sheep to avoid abortion and foetal teratology.
Inactivated vaccines are normally used in non-endemic RVF countries.
MP12: The mutagen-derived MP12 candidate vaccine virus generated after 12 passages of the parental ZH548 strain (Egypt 1978) in the presence of the mutagen 5’-flurouracil was shown to be safe and effective for use in pregnant sheep and cattle, and has even been inoculated directly into bovine foetuses without apparent deleterious effects. However, the experiments were conducted with animals beyond the first trimester of pregnancy, after organogenesis has occurred when foetuses are less susceptible to the teratogenic effects of attenuated viruses. Teratology occurred in 14 per cent of lambs of sheep inoculated at an earlier stage of pregnancy. However, the MP12 vaccine has proven to be a safe and effective animal and human vaccine among non-pregnant individuals and recently was successfully tested in small scale Phase I/II human clinical trials.
Clone 13 vaccine: It is a live-attenuated vaccine based on a naturally attenuated strain (RVFV 74HB59) that was isolated in the Central African Republic from a RVF patient. It lacks 69 per cent of the NSs gene, a major virulence factor of the virus. Available data indicate that the Clone 13 vaccine did not cause detectable viraemia in vaccinated ruminants, thus minimizing the risk of virus transmission to the foetus or to mosquitoes and genome segment reassortment events with wild- type viruses. However, a recent report indicated that Clone 13 virus could cross the ovine placental barrier and be associated with foetal infections, malformations, and stillbirths when administered in an overdose to pregnant ewes at 50 days of gestation. Nevertheless, the Clone 13 vaccine at the recommended dose is considered to be safe and efficacious in domestic ruminants.
Challenges to vaccination acceptance
It is advisable in African countries with large sheep and goat populations to immunize the offspring of vaccinated ewes and nannies on a regular basis at six months of age, when colostral immunity has waned, with a single dose of the modified live Smithburn vaccine. This should afford life-long protection. Lambs and kids of susceptible unvaccinated dams can be immunized at any age.
Epidemics of RVF tend to occur at irregular intervals of many years, and it is usually difficult to persuade farmers to vaccinate livestock during long inter-epidemic periods when no apparent losses are occurring to justify vaccination costs. Compounding the sometimes long inter-epidemic periods is that the occurrence of epidemics is difficult to predict and outbreaks typically have a very sudden onset. Ideally, in African countries where RVF is endemic, large sheep and goat populations should be immunized routinely. The offspring of previously vaccinated livestock could be vaccinated on a regular basis at six months of age, when colostral immunity has waned, with a single dose of a modified live vaccine. Typically, this class of vaccines should provide life-long protection.
No RVF vaccine has been authorised for use in the EU Member States: only emergency vaccination is permitted after authorization and following proper EU procedures.
Vaccination of at-risk humans
It is essential that humans living in endemic areas and especially veterinarians and others engaged in the livestock industry should be made aware of the potential dangers of exposure to zoonotic agents in handling tissues of diseased animals, and that biosafety and biosecurity precautions should be increased during RVF epidemics. Currently only one human vaccine is approved for emergency use in humans. It is a formalin-inactivated cell culture vaccine (TSI-GSD 200) produced in the USA that is used only to immunize laboratory and field workers who are regularly exposed to RVF infection such as during outbreaks.
Recently, efforts to promote the development of licensed RVFV vaccines for humans has gained momentum. With this renewed prioritization it is highly likely that the further development of next-generation vaccines for humans will be achievable in the near future.
Surveillance and forecasting of outbreaks
Surveillance can be planned with different objectives and approaches and can include forecasting, early warning, raising awareness, outbreak investigations, environmental surveillance, vector surveillance and sentinel herd monitoring.
Three surveillance methods for RVF, namely, syndromic surveillance, participatory surveillance and risk-based surveillance are recommended by the WOAH. The focus of syndromic surveillance is on the detection of clinical disease such as abortions, mortality in livestock and wildlife particularly in newborn and young lambs, kids or calves especially during favourable weather conditions conducive for vector transmission of RVFV. Participatory surveillance focuses on how best animal owners can be helped to identify and solve their health problems and to better understand the risks of RVF. Risk-based surveillance is the surveillance of locations, populations, and periods with increased disease threats with the aim to improve disease detection rapidly and therefore better use of resources. Risk-based surveillance uses quantitative or qualitative information that helps in disease control.
Sentinel herds are particularly useful to detect inter-epidemic transmission of RVFV. The activities at sentinel sites should include clinical observations and serological monitoring of herds, measurement of rainfall and other climatological parameters and vector sampling and surveillance.
Recently, epidemiological models have become available to investigate the various aspects of the epidemiology of RVF. with the aim to get a better understanding of the factors at play among vectors, hosts and the environment. Predictive models have been developed to act as early warning systems for RVF outbreak occurrence so that surveillance systems can be set up to detect RVFV circulation in mosquitoes and livestock.
Attempts have been made to utilize satellite imaging to predict RVF outbreaks through the development of green vegetation indices or through monitoring of southern Pacific and Indian Ocean surface temperatures.
New approaches for the establishment of early warning systems for RVF should include monitoring of climatic data of different environments.
Climate change and extreme weather events during the last decades may support the expansion of the geographical range of RVF northwards and into the Mediterranean countries. Forecasting models and livestock trade/movement data] may be useful in future to predict outbreaks of RVF outbreaks.
Figures
from: Coetzer JAW, Paweska JT, Bird B, Swanepoel R, Odendaal L, Fafetine J. Rift Valley fever. Anipedia. Published online May 31, 2021.
Registration to Anipedia is free of charge. Sign up for the full text https://anipedia.org/resources/rift-valley-fever/1167